Analytical Ultracentrifugation (AUC) for Characterization of Lipid Nanoparticles (LNPs): A Comprehensive Review
Introduction
Lipid nanoparticles (LNPs) and liposomes (Figure 1) have revolutionized the medical field by serving as carriers for a wide range of therapeutic molecules, and have been used for cancer treatments, drug delivery, and vaccine development, including the recent COVID-19 mRNA vaccines by Moderna and Pfizer-BioNTech. mRNA cannot be injected directly into a patient due to its immunogenicity, toxicity, and susceptibility to RNase degradation and renal clearance;1 however, by packaging the RNA into LNPs, these issues can be overcome. LNPs offer additional advantages, such as improved stability, targeted delivery, and adaptability to changing viral strains.2
The biophysical characterization of LNPs is crucial for assessing their quality, efficacy, and safety. The accurate determination of size and homogeneity of LNP formulations is essential, as recent studies in model systems have demonstrated that they may influence the immunogenicity and potency of the treatment;3 however, determining the accurate size distribution of an LNP formulation is difficult due to their inherent heterogeneity. Although dynamic light scattering (DLS) is commonly used for size determination, its measurements are based on Brownian motion, which limits the upper range of detection and, therefore may miss aggregates.4 Additionally, DLS cannot differentiate between empty and loaded particles. To address these challenges, the FDA recommends employing orthogonal techniques for measurement.5 Other important parameters to characterize include the free and bound/encapsulated cargo, the drug copy number distribution, the empty/full ratio of nanoparticles, and their stability.

![]() |
Ionizable neutral lipid |
![]() |
Ionizable cationic lipid |
![]() |
´Helper´ lipid |
![]() |
Cholesterol |
![]() |
PEG-lipid (polyethylene glycol lipid) |
![]() |
Nucleid acid (e.g. mRNA) |
Figure 1: LNPs are small particles used in the pharmaceutical and biotechnology industries to help improve drug delivery. They are composed of a lipids which encapsulate the nucleic acid or other therapeutic agent, allowing for improved cell targeting and enhanced drug efficiency.
Analytical ultracentrifugation (AUC) is a technology gaining traction for LNP characterization. When the samples are subjected to centrifugal forces, they are hydrodynamically separated based on their sedimentation coefficient (resulting from the analyte mass and density) and diffusion coefficient (resulting from particle shape). For LNPs, this can result in either sedimentation or flotation (Figure 2), depending on the lipid composition and cargo load. During centrifugation, an analyte’s sedimentation/flotation and diffusion patterns are measured by tracking their absorption properties. From the measured sedimentation and diffusion parameters, size distributions, cargo loading, molar mass, and more can be determined for these challenging systems.
This review will examine how AUC has been used to characterize LNPs, and how it compares to other methodologies. Additionally, from these studies, it does not appear that the gravitational force generated during centrifugation affects the LNPs; if it did, this would be identifiable during analysis.6
A)
B)
Figure 2: Examples of sedimenting and floating data collected via AUC: Examples of the boundary shape of particles during centrifugation in the AUC. The earlier scans are depicted in purple and later scans in blue, then green. A) Depicts a sedimenting particle. B) Depicts a particle that floats during the centrifugation process.
Several studies have used AUC to determine size and size distribution of different LNP formulations, including siRNA, mRNA, and doxorubicin encapsulating systems.5–7 These studies compared the average size and size distributions determined by AUC to techniques such as dynamic light scattering (DLS), nanoparticle tracking analysis (NTA), transmission electron microscopy (TEM), and asymmetrical-flow field-flow fractionation (AF4) in combination with multi-angle light scattering (MALS). The studies found that the average size determined by AUC corroborated well with all methods tested. Further, AUC could accurately determine the LNP size distributions for all formulations in agreement with AF4-MALS and Cryo-TEM. AF4-MALS and AUC provided high resolution when measuring and detecting samples with multiple polydisperse and high molecular weight species5,8 (Figure 3). This is due to the ability of both methods to combine a separation technique and in-process detection. AUC also adds an additional dimension by separating the molecules based on size and density, resulting in accurate size distribution determinations for LNP samples.
Moreover, AUC has been used to study the free and bound cargo present in formulations,6,7 which is a critical parameter, as free cargo could result in toxicity and increased immune reactions.9 The Optima AUC analytical ultracentrifuge contains a light source that can measure up to ~20 wavelengths between 190 – 800 nm in a single experiment. With this capability the adsorption of the cargo (e.g., 260 nm for nucleic acids and 490 nm for Doxil) can be measured throughout the experiment. The LNP signal can also be detected, however, because lipids do not absorb light, the signal measured is the scattered light from the LNPs. The scattering signal can typically be detected between 215-280 nm, depending on the size of the LNP. It should be noted that the scattering signal from the LNP will scale differently from the adsorption signal detected from the cargo.6 By detecting the sample’s sedimentation and diffusion patterns throughout the experiment, Mehn et al. calculated the amount of free drug present, and their results aligned well with HPLC and DLS measurements.7 Henrickson et al. performed multiwavelength and fluorescence detection methods to show that their siRNA LNPs contained only encapsulated RNA.6
A) Batch DLS
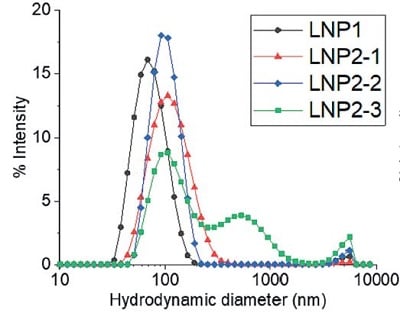
B) MADLS
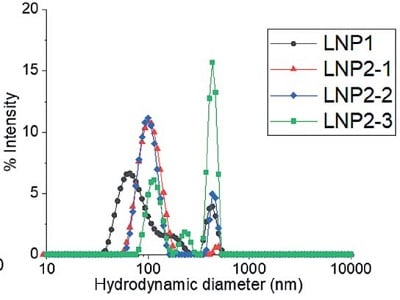
C) Batch NTA
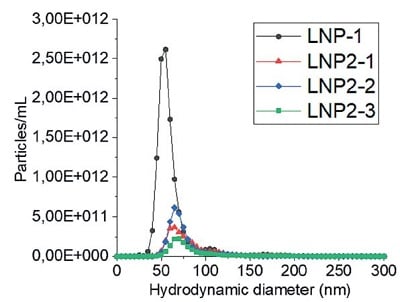
D) AUC
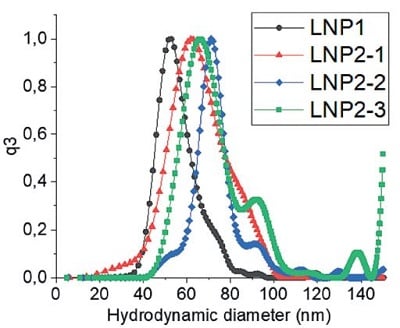
Figure 3: Hydrodynamic diameter of LNP formulations: Hydrodynamic diameter determination for four different LNP formulations measured by A) batch DLS, B) MADLS (multi-angle DLS), C) Batch NTA, and D) AUC. For more information and for an interpretation of the reference colors in the figure, see https://pubmed.ncbi. nlm.nih.gov/38253203/ Parot et al. DOI: 10.1016/j.jconrel.2024.01.037, Epub 2024, https://creativecommons.org/licenses/by/4.0/, image was not altered.
It is still unclear what role empty LNPs might play when or if they are administered during drug treatments; however, their characterization could help improve LNP production and ensure safe therapies. Using density matching AUC, where a sample is measured multiple times in buffers of different densities, it is possible to determine the density distribution of the entire sample.5–7 Once the density distribution of the sample is known, it can be compared to an empty LNP sample. If an overlap in density is present, this could indicate that the sample contains a percentage of empty LNPs. Bepperling and Richter built on this method and used it to calculate the number of mRNA copies per capsid.10 They found that their mRNA LNP formulation had a hydrodynamic radius distribution between 25 – 100 nm, and that it contained between 1 – 10 mRNA copy numbers per capsid. They determined that this single-digit value was plausible and in line with results from other studies of similarly sized LNPs.11–13 These studies highlight the ability of AUC to characterize empty-full LNP distributions and mRNA payload capacity. Both are important parameters to consider, as they could impact cellular activities and mRNA expression kinetics,12 and can help optimize LNP production and delivery of a wider range of therapeutics. Finally, the stability of LNP formulations must be assessed at different time points while treating the samples according to conditions that will occur during real-life applications, such as freeze/thaws and manipulation at room temperature.9 Thaller et al. compared AUC and DLS to characterize LNP polydispersity and stability under different stress conditions.8 DLS could qualitatively determine the hydrodynamic radius and identify changes in the formulations when exposed to freeze/thaw and mechanical stress, but not heat stress, at 50°C. They determined that AUC was a quantitative characterization method for LNPs that could provide more precise particle size distributions, identify changes in all tested stress conditions, and observe changes in particle density, which DLS cannot detect.
These studies highlight the versatility and utility of AUC for the characterization of LNP formulations. AUC can precisely determine the size distribution of LNP formulations in agreement with AF4-MALS and TEM. In addition, it can identify and quantify the presence of free cargo and empty LNPs in solution and can be used to determine the number of mRNA copies per LNP. Overall, AUC is a quantitative, first-principle method that is non-destructive, provides a comprehensive and reliable approach to the characterization of LNPs, and has become an indispensable tool in LNP research.
References
- Yin H, Kanasty RL, Eltoukhy AA, Vegas AJ, Dorkin JR, Anderson DG. Non-viral vectors for gene-based therapy. Nat Rev Genet. 2014 Aug;15(8):541–55.
- Corbett KS, Edwards DK, Leist SR, Abiona OM, Boyoglu-Barnum S, Gillespie RA, et al. SARSCoV- 2 mRNA vaccine design enabled by prototype pathogen preparedness. Nature. 2020 Oct;586(7830):567–71.
- Chen L, Ge B, Casale FP, Vasquez L, Kwan T, Garrido-Martín D, et al. Genetic drivers of epigenetic and transcriptional variation in human immune cells. Cell. 2016 Nov 17;167(5):1398-1414.e24.
- Caputo F, Vogel R, Savage J, Vella G, Law A, Della Camera G, et al. Measuring particle size distribution and mass concentration of nanoplastics and microplastics: addressing some analytical challenges in the sub-micron size range. J Colloid Interface Sci. 2021 Apr 15;588:401–17.
- Parot J, Mehn D, Jankevics H, Markova N, Carboni M, Olaisen C, et al. Quality assessment of LNP-RNA therapeutics with orthogonal analytical techniques. J Control Release. 2024 Mar;367:385–401.
- Henrickson A, Kulkarni JA, Zaifman J, Gorbet GE, Cullis PR, Demeler B. Density Matching Multi-wavelength Analytical Ultracentrifugation to Measure Drug Loading of Lipid Nanoparticle Formulations. ACS Nano. 2021 Mar 23;15(3):5068–76.
- Mehn D, Iavicoli P, Cabaleiro N, Borgos SE, Caputo F, Geiss O, et al. Analytical ultracentrifugation for analysis of doxorubicin loaded liposomes. Int J Pharm. 2017 May 15;523(1):320–6.
- Thaller A, Schmauder L, Frieß W, Winter G, Menzen T, Hawe A, et al. SV-AUC as a stability-indicating method for the characterization of mRNA-LNPs. Eur J Pharm Biopharm. 2023 Jan;182:152–6.
- Guerrini G, Magrì D, Gioria S, Medaglini D, Calzolai L. Characterization of nanoparticles-based vaccines for COVID-19. Nat Nanotechnol. 2022 Jun 16;17(6):570–6.
- Bepperling A, Richter G. Determination of mRNA copy number in degradable lipid nanoparticles via density contrast analytical ultracentrifugation. Eur Biophys J. 2023 Jul;52(4–5):393–400.
- Sabnis S, Kumarasinghe ES, Salerno T, Mihai C, Ketova T, Senn JJ, et al. A Novel Amino Lipid Series for mRNA Delivery: Improved Endosomal Escape and Sustained Pharmacology and Safety in Non-human Primates. Mol Ther. 2018 Jun 6;26(6):1509–19.
- Li S, Hu Y, Li A, Lin J, Hsieh K, Schneiderman Z, et al. Payload distribution and capacity of mRNA lipid nanoparticles. Nat Commun. 2022 Sep 23;13(1):5561.
- Carrasco MJ, Alishetty S, Alameh M-G, Said H, Wright L, Paige M, et al. Ionization and structural properties of mRNA lipid nanoparticles influence expression in intramuscular and intravascular administration. Commun Biol. 2021 Aug 11;4(1):956.