A
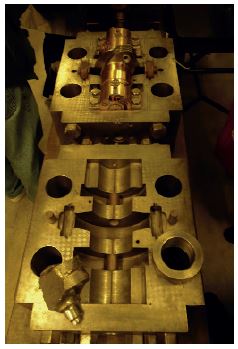
Analytical ultracentrifugation (AUC) has a rich history that dates back to the early 20th century. The technique was pioneered by Swedish chemist Theodor Svedberg, who was awarded the Nobel Prize in Chemistry in 1926 for his innovative work on dispersed systems, particularly colloids. His development of the first analytical ultracentrifuge facilitated the determination of molecular weights and the study of the sedimentation behavior of macromolecules.1 Since then, AUC has experienced significant advancements in instrumentation and software, making it an indispensable tool in molecular biology, biochemistry, pharmacology, material science, synthetic polymer chemistry and various other fields.
The first commercially available AUC was the Model E analytical ultracentrifuge, released in 1947 by SpinCo, who partnered with and became a new division of Beckman Instruments in 1955. The Model E was used primarily in molecular biology and biochemistry to determine protein molecule weight.2 However, in the years that followed, the AUC started to fall out of favor due to the complexity of maintaining the instrument, challenges with data analysis, and the eventual need for more quantitative results.
Technological innovations in the 1970s and 1980s, such as digital data acquisition and new rotor designs, resulted in Beckman Coulter releasing, in 1991, the XL-A Analytical Ultracentrifuge equipped with a UV-vis detector, and, in 1996, the Optima XL-I analytical ultracentrifuge equipped with both UV-Vis and Rayleigh interference detectors. The company later renamed these instruments the ProteomeLab XL-A and XL-I analytical ultracentrifuges. These innovations enhanced the accuracy and efficiency of AUC experiments and expanded their range of applications.
Then, in 2016, Beckman released the Optima AUC analytical ultracentrifuge, which introduced several improvements to enhance its capabilities and ease of use. Some of these improvements include the capability of multiwavelength experiments, which enables precise analysis of complex systems at discrete wavelengths, temperature control from 4-40°C, remote monitoring capabilities, which enable set up, monitoring, and extraction of data from virtually any location, improved signal to noise, and increased scan speeds.
Throughout its history, AUC has proven to be a versatile and valuable technique for understanding the fundamental properties of macromolecules in solution. Over the years, several review articles about using AUC have been published, highlighting its capabilities.3–7 Here, we will highlight a few examples of recent publications that leveraged the Optima AUC to explore various aspects of protein research, including membrane proteins, antibodies, protein-nucleic acid interactions and viral vectors.
A
B
C
D
Figure 1: AUC over the years: After the development of (a) the original AUC by Svedberg, there have been several commercially available AUCs, including (b) the Model E, (c) the ProteomeLab XL-I, and (d) the Optima AUC.
* when ambient temperature is < 25°C
Since its inception, AUC has been a foundational technique for the characterization of proteins and protein therapeutics.8 When developing protein therapeutics such as antibodies, it is critical to minimize the formation of aggregates, as they can reduce drug efficacy and potentially trigger immune responses.9 It is vital, therefore, to carefully characterize oligomers and aggregates that might be present in the solution. Size exclusion chromatography (SEC) is commonly used for aggregate quantification in development and quality control, but its limitations, such as sample dilution, potential dissociation of aggregates, buffer considerations, and interactions with the column matrix, necessitate the use of orthogonal techniques such as AUC for more accurate characterization.9–12 Sedimentation velocity AUC (SV-AUC) is particularly valuable for quantifying biotherapeutic aggregates and oligomers, as it differentiates species based on hydrodynamic properties without the confounding effects of stationary phases.
The Optima AUC uses two detection methods: UV-Vis absorbance and Rayleigh interferometry, which can be used separately or simultaneously to measure radial concentration as species sediment within the instrument. When using UV-Vis absorbance optics, targeting a concentration between 0.3 – 1 optical density (OD) at the wavelength measured is typically recommended. The most commonly used centerpiece has a 12 mm pathlength; however, a centerpiece with a 3 mm pathlength can be used to measure higher concentrations. Additionally, researchers typically use wavelengths in the 215-230 nm range if low-protein concentrations need to be measured. However, in this range, the OD change between wavelengths is steep; due to this, a shift in the measured wavelength could lead to erratic results. This is especially problematic with the older ProteomeLab AUCs, which had a wavelength accuracy specified at 4 nm.14,15 The Optima AUC contains improved absorbance optics, with the wavelength accuracy specified at 0.5 nm. This provides confidence in the agreement of the set and actual wavelengths, resulting in accurate and reliable results. In cases where much higher concentrations are required or the measured particles do not absorb, the Rayleigh interference optics can be used. The interference detection optics measures the differential refractive index of the solution compared to the reference solution and has allowed researchers to measure monoclonal antibodies up to ~45 mg/mL.13
Although SV-AUC has some limitations for protein therapeutic characterization, including lower throughput, it remains a preferred method for aggregate characterization in monoclonal antibody (mAb) formulations.9 The Optima AUC also allows for improved throughput by enabling researchers to measure a sample in both channels of the AUC cell when measuring in intensity mode with the UV-Vis optical system. Bou-Assaf, G., et al. have created a comprehensive guide to overcome some of the issues that can be encountered, and help with the design, execution and analysis of aggregates in protein therapeutic preparations with a focus on mAbs.9
Another highly studied area of research is membrane proteins, which are involved in various cell processes and regulations. Purification of these proteins is challenging, however, with the bottleneck frequently being the stability of the membrane proteins in detergents.16 Li, D., et al. employed SV-AUC to quantitatively assess the behavior of their membrane protein, TmrA, under different detergent conditions, offering insights into optimizing membrane protein purification.16 By combining UV-Vis absorbance and Rayleigh interference detection, they characterized the protein’s different conformational and aggregation states based on its sedimentation coefficients in various detergent concentrations, and optimized the step at which high detergent concentrations could be added (Figure 2).
A
B
C
D
Figure 2: The membrane protein TmrA’s sedimentation coefficient when purified or supplemented with DDM at various concentrations. A and B show the pairwise comparison results for the UV-vis detector for TrmA at two different concentrations. C and D show the pairwise comparison results from the interference detector. For more information, please see Li et al. 2021 doi: 10.3390/membranes11100780, https://creativecommons.org/licenses/ by/4.0/ images were not altered.
Protein nucleic acid interactions are another area of research that benefits from the characterization powers of AUC, especially since the addition of multiwavelength (MW) capabilities in the Optima AUC. Multiwavelength AUC has been pivotal in understanding the stoichiometry and thermodynamics of protein-nucleic acid interactions. It allows for the decomposition of analytes in solution based on their hydrodynamics and spectral properties to determine their molar quantities and stoichiometry, thus offering a more comprehensive characterization of their interactions.17 In a notable study, Horne, C., R., et al., used MW AUC to aid a functional understanding of how transcriptional regulators mediate the regulation of genes in bacteria as a response to environmental changes.18 To do so, it was essential to validate the stoichiometry involved in the protein–DNA binding to gain insight into the thermodynamics of these interactions. By hydrodynamically separating the interacting and non-interacting analytes due to the centrifugal force, and optically separating the spectral signals from the protein and the DNA, the researchers determined the molar concentrations of the analytes at each sedimentation coefficient. This revealed that three dimers of their protein, NanR, sequentially bind to their DNA repeat operator with low nanomolar affinity (Figure 3). This experiment and several others,17,19–21 underscore the added experimental knowledge that can be obtained through multiwavelength AUC, highlighting its invaluable role in advancing our understanding of complex biological interactions.
A
B
C
D
E
Figure 3: Optically deconvoluted multiwavelength AUC interaction study of NanR and DNA. (a) The sedimentation coefficient distributions of the controls NanR (blue) and the DNA (black) were measured at 280 and 260 nm, respectively. (b-e) Show the sedimentation coefficient distributions from the titration of increasing concentrations of NanR into the DNA, resulting in a shift in sedimentation coefficient consistent with complex formation. The shaded areas highlight heterocomplexes, and each heterocomplex‘s molar ratio is shown. For more information, please see Horne et al. 2021. Doi: 10.1038/s41467-021-22253-6, https://creativecommons.org/licenses/by/4.0/ images were not altered.
Finally, AUC has seen significant adoption in the characterization of viral vectors used for drug delivery, particularly adeno-associated viruses (AAVs).22 The safety and efficacy of AAV treatments can be compromised by quality issues such as empty or partially filled capsids or other contaminants such as endotoxins, residual host cell DNA, and defective particles, which can affect large-scale production and patient safety.23 Due to AUC’s ability to fractionate particles in solution based on their size, density, and shape during the detection, it can characterize several attributes of AAVs, including their sedimentation coefficients, the percentage of loaded, empty and partially filled capsids, and the presence of other contaminants (Figure 4).24,25
A
A
B
C
Figure 4: Experimental data highlighting AUC capabilities to differentiate AAV loading states. (a) Graphical explanation of the combination of different capsid (ncapsid) and full-length ssDNA (nssDNA) on the left and the theoretically correlated sedimentation coefficient on the right. Correlation plot between the number of nucleotides per capsid and s-value for each association state of particles (right). (b) Highlights the author‘s ability to calculate the number of nucleotides in an AAV capsid using multiple regression analysis with each component‘s molar extinction coefficient spectra. For more information, please refer to the publication. (c) Sedimentation velocity results for an AAV1 sample, highlighting empty, partially filled, full, and aggregated capsids. The aggregated capsid illustration is a representation of possible components. For more information, please see Maruno et al. 2021.doi: 10.1016/j. xphs.2021.06.031, https://creativecommons.org/licenses/by/4.0/ images were not altered.
Analytical ultracentrifugation remains a versatile and valuable technique for understanding the fundamental properties of macromolecules in solution. The continuous advancements in AUC technology demonstrate its indispensable role in various fields of research. The most recent AUC instrument, the Optima AUC, introduced improvements such as enhanced wavelength accuracy, allowing more confidence in your measurements. This is especially useful when measuring low-concentration proteins in the 215-230 nm range, where the OD change is steep between wavelengths. Faster scanning capabilities enable the collection of dense raw datasets, which can help improve RSMDs and be useful when collecting information on fast sedimenting analytes. Multiwavelength capabilities, which allow for protein and DNA overlapping signals to be deconvoluted into separate profiles based on their spectral differences, provide direct access to the molar stoichiometry of interacting complexes and improve the characterization of viral vector loading states. Increased throughput can also be achieved when measuring with the UV-Vis detector system, as both channels can be used for samples when measuring in intensity.* Here, we highlighted select publications that demonstrate the strengths of the Optima AUC. From optimizing protein purification to characterizing complex interactions and ensuring the quality of gene therapy and drug delivery vectors, AUC continues to provide critical insights that drive scientific progress. Through the review of recent studies, this paper highlights the enduring strengths and evolving capabilities of AUC in modern scientific research.
* The higher concentrated sample should still be loaded into the sample channel (Channel A).